The Economic Feasibility of Geoengineering Solutions
Clinton Kaboni and Lauren Viesner
Introduction
This commentary offers a comprehensive examination of the economic dimensions of geoengineering, focusing specifically on the contrasting strategies of carbon dioxide removal (CDR) and solar radiation management (SRM) discussed by Harding and Moreno-Cruz (2019) and the economics of geoengineering. Their analysis goes into each approach’s distinct methods and impacts and highlights the cost-effectiveness and timescales associated with their implementation.
Carbon Dioxide Removal vs. Solar Radiation Management
Within the context of geoengineering, CDR involves removing and storing CO2 in the atmosphere, while SRM attempts to reduce global temperatures by reflecting solar radiation (Harding & Moreno-Cruz, 2019). Because of their innate differences, they both involve differing methods of controlling climate; thus, their cost and timescales also vary. Harding and Moreno-Cruz discussed that between the two strategies, SRM exhibits lower cost and its impact on climate change is observed within months rather than decades. In contrast, SRM is seen as the opposite, demonstrating high cost and being much slower to impact. However, SRM is limited, cannot address all climate change, and may even introduce more problems in the future. As for CDR strategies, while they are much slower and expensive, they perfectly counteract climate change. However, the cost and speed of this strategy are comparable to traditional mitigation.
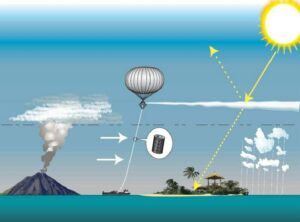
For SRM, the cheapest technique is stratospheric aerosol albedo modification, which reduces the radiative forcing of sulphate particles released into the stratosphere. This could be done using current technology, such as releasing these particles using naval rifles, airplanes, balloons, or constructing large towers (Harding & Moreno-Cruz, 2019). Robock et al. (2019) state that the cost for these different methods of injecting 1 Tg of sulphur gas per year into the stratosphere ranges from 1.2 to 30 billion dollars a year. This cost range depends on the technology used and the preferred particle levels. (Harding & Moreno-Cruz, 2019).
With CDR, many techniques may be utilized, with direct air capture (DAC) being mentioned the most in the literature, which is when CO2 is directly taken from the air and then stored. The cost of this technique varies considerably; Harding and Moreno-Cruz (2019) write that initial estimates for direct air capture at an industrial level find implementation costs of around USD 30 to 100/tCO2. Follow-up research incorporating the innovation of technologies, learning, and commercial expansion finds higher costs ranging from USD 200/tCO2 to as high as USD 1,000/tCO2 initially, but also finds they can fall to between USD 30/tCO2 and USD 300/tCO2 later in the century. According to Harding and Moreno-Cruz, many researchers have concluded that direct air capture expansion may be feasible; however, once their high costs decrease from further advancements, it will continue to have a limited impact on climate change.
This commentary acknowledges Scheider’s (2008) concerns about geoengineering feasibility, especially regarding international cooperation and governance challenges. Scheider highlights potential conflicts due to differing ideologies, emphasizing the need for transparent and fair governance. These challenges and economic considerations raised by Harding and Moreno-Cruz (2019) underscore the complexities of geoengineering strategies.
Table 1 summarises how CDR compares to SRM.
Table 1: Comparing Carbon & Solar Geoengineering
Skip Table 1 | ||||
Methods | Description | Cost | Benefits | Risks/Costs |
---|---|---|---|---|
Carbon Dioxide Removal (CDR) | Seeks to directly remove carbon dioxide, generated from economic activity using fossil fuels and land use changes, from the atmosphere. Globally, we emit 40 gigatonnes a year. | Costs can be high, particularly for large scale implementation.
$30 up to $1,000 per tonne of CO2. |
|
|
Solar Radiation Management (SRM) | Seeks to reflect a small fraction of sunlight into space or increase the amount of solar radiation that escapes back into space to cool the planet. | Costs 1.2 to 30 billion dollars a year. It varies depending on the method, but some solar geoengineering techniques may be relatively inexpensive compared to traditional mitigation strategies. |
|
|
Note. Data from Burrow (2018), Corry (2017), Faran & Olsson (2018), Gunderson et al. (2018), Harding & Moreno-Cruz (2019), Harding et al. (2020), Robock et al. (2019), Scheider (2008), and Tollefson (2018).
Conclusion
While geoengineering may seem like a tempting solution to mitigate the effects of climate change, it is fraught with uncertainties and risks, especially given the current difficulty in coordinating a response to climate change. CDR and SRM offer climate risk mitigation alternatives. More research on the impacts and damages from climate engineering is needed to inform policy decisions and ensure responsible implementation, considering its environmental, climatic, and societal implications. Ultimately, investing in mitigation measures and exploring alternative methods to reduce our impact on the Earth should remain paramount as we navigate the challenges of climate change.
Media Attribution
Figure 1: “SPICE SRM overview” by Hughhunt (2011), via Wikimedia Commons, is used under a CC BY-SA 3.0 license.
References
Burrow, L. (2018, November 28). Calculating solar geoengineering’s technical costs. Harvard John A. Paulson School of Engineering and Applied Sciences. https://seas.harvard.edu/news/2018/11/calculating-solar-geoengineerings-technical-costs.
Corry, O. (2017). The international politics of geoengineering: The feasibility of Plan B for tackling climate change. Security Dialogue, 48(4), 297–315. https://doi.org/10.1177/0967010617704142.
Faran, T., & Olsson, L. (2018). Geoengineering: Neither economical, nor ethical—a risk–reward nexus analysis of carbon dioxide removal. International Environmental Agreements: Politics, Law and Economics, 18, 63–77. https://doi.org/10.1007/s10784-017-9383-8.
Gunderson, R., Petersen, B., & Stuart, D. (2018). A critical examination of geoengineering: Economic and technological rationality in social context. Sustainability, 10(1), Article 269. https://doi.org/10.3390/su10010269.
Harding, A., & Moreno-Cruz, J. B. (2019). 25 – The economics of geoengineering. In T. M. Letcher (Ed.), Managing global warming: An interface of technology and human issues (pp. 729–750). Academic Press.
Harding, A. R., Ricke, K., Heyen, D., MacMartin, D. G., & Moreno‐Cruz, J. (2020). Climate econometric models indicate solar geoengineering would reduce inter-country income inequality. Nature Communications, 11, Article 227. https://doi.org/10.1038/s41467-019-13957-x.
Hughhunt. (2011). SPICE SRM overview [Image]. Wikimedia Commons. https://commons.wikimedia.org/wiki/File:SPICE_SRM_overview.jpg
Robock, A., Marquardt, A., Kravitz, B., & Stenchikov, G. (2009). Benefits, risks, and costs of stratospheric geoengineering. Geophysical Research Letters, 36(19). https://doi.org/10.1029/2009GL039209.
Schneider, S. H. (2008). Geoengineering: Could we or should we make it work? Philosophical Transactions of the Royal Society A, 366(1882), 3843–3862. https://doi.org/10.1098/rsta.2008.0145.
Thompson, C. (n.d.). Proposed methods for climate intervention… [Image]. Climate Program Office. https://cpo.noaa.gov/atmospheric-aerosols-and-their-potential-roles-in-solar-climate-intervention-methods/.
Tollefson, J. (2018, June 7). Sucking carbon dioxide from air is cheaper than scientists thought. Nature. https://doi.org/10.1038/d41586-018-05357-w.