Satellite Technology, Methane, & Climate Change Economics
Patrick Izett
Introduction
Unlike CO2, atmospheric methane (CH4) concentrations have risen faster than ever in the past two decades and, since 2014, are now approaching the most greenhouse-gas-intensive scenarios (Saunois et al., 2016). CH4 is responsible for roughly 30% of the rise in global temperatures since the Industrial Revolution, so the need for immediate reductions in CH4 emissions is imperative to limiting global warming and improving air quality (International Energy Agency [IEA], 2023). However, with advancements in satellite imaging, the ability to map global CH4 leaks in real-time has become increasingly more accessible. The UN Environment Programme estimated that the global cost of adapting to climate impacts is expected to grow to $140-300 billion per year by 2030 and $280-500 billion per year by 2050, with the estimated costs of damages from warming in 2100 for 1.5 C and 2 C were $54 trillion and $69 trillion (Black, 2014).
Using Satellite Technology to Combat Climate Change
Satellite technology is at the forefront of the advancements made in combating climate change, with these satellites able to capture information in real-time. We can distinguish three major parameters that help us characterize satellite observations: spectral resolution, spatial resolution, and temporal resolution (Olczak et al., 2020). Furthermore, these satellites provide “hyper-spectral images in a short-wave infrared (SWIR) spectrum, in which CH4 is a strong absorber (Ehret et al., 2022). Traditional methods for mapping CH4 struggle to identify key areas around the globe. Surface stations located in South America, Central Africa, Southeast Asia, and Boreal Eurasia, all of which are key regions for CH4 emissions, where these traditional methods are unable to accurately identify CH4 leaks (Pison et al., 2013). Currently, satellite technology detects CH4 plumes using Tropospheric Monitoring Instrument sensors that can identify source locations by longitude and latitude (Kayrros, n.d.).
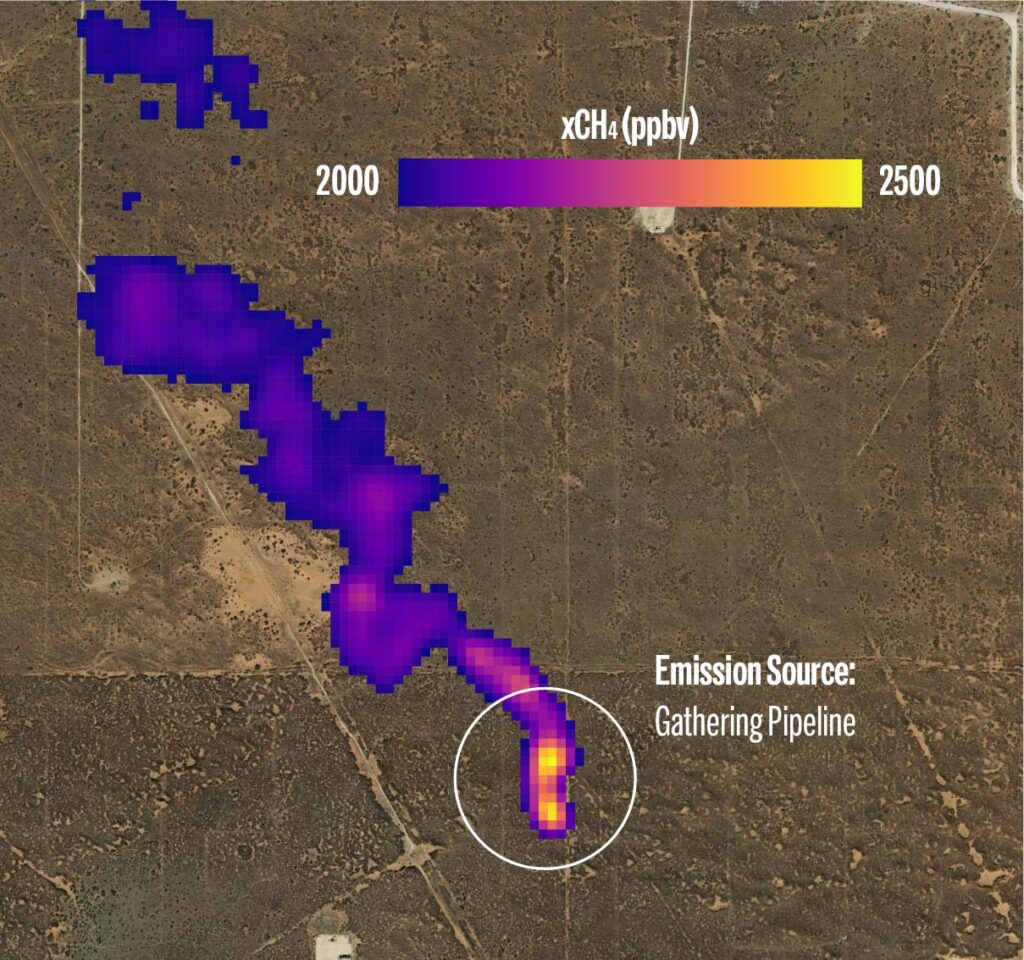
Reducing Methane Leaks
According to economic theory, companies will capture methane emissions if the economic costs of doing so are less than the value of the lost gas (Hausman & Raimi, 2019). While there are some economic incentives to prevent methane leaks, there are not enough. From an outside perspective, the damage caused by each additional MMBtu of methane emissions ranges from $2.80 to $27 of value lost from gas in the U.S. (Hausman & Raimi, 2019). In addition to the direct costs, the indirect costs of methane leaks affect agricultural productivity through ozone and climate change. Recent studies have found evidence of consequences to health and agricultural damage to be larger than previously believed. Most identified methane abatement controls cost less than the societal benefits of USD 4,300 per tonne of methane. Reducing these CH4 emissions would equate to a global saving of roughly USD 470 billion (Climate and Clean Air Coalition [CCAC] & United Nations Environment Programme [UNEP], 2021).
Advantages
The key advantage of utilizing satellite technology to detect CH4 plumes is the ability to observe the vast diversity of CH4-emitting sectors. Plumes from different sectors have different shapes and sizes (Ayasse et al., 2019). For example, satellites can infer different trends in CH4 mapping, providing data on source types such as oil and gas, livestock, and wetlands. Case studies by Cusworth et al. (2018) and Pandey et al. (2019) further illustrate the use of satellite tech and its economic viability. Each study uses TROPOMI observations to discover different plumes of CH4 in the U.S. (Nisbet et al., 2020). Highlighting these leaks allows for real-time action and the ability to map where attention is needed. With economic incentives to innovate and continually abate methane, satellite technology and data collection are at the forefront of addressing methane emissions (Olczak et al., 2020).
Furthermore, satellite technology can aid in the performance and analysis of the marginal abatement cost (MAC). The MAC shows the added costs of achieving a 1-unit decrease in the emission level. Table 1 shows eight sources of potential abatement and marginal abatement costs for the Canadian national aggregate methane. The first 7.5 million tonnes can be reduced at a marginal benefit using gas capture technology, electric pump and instrument air. Reductions after 7.5 million tonnes of marginal abatement costs are positive. Furthermore, old technologies have a higher MAC for any given reduction of emission than newer, cleaner technologies. Therefore, employing new satellite technology will aid in MAC and the lowering of methane emissions around the globe.
Table 1: Potential Abatement & Marginal Costs for Canadian National Aggregate Methane
Skip Table 1 | ||
Source | MAC ($/tonne CO2e) | kTonnes CO2e Reduced |
---|---|---|
Compressor Blowdowns – Gas Capture | -12 | 2,500 |
Kimray Pumps – Electric Pump | -11 | 5,000 |
High Bleed Pneumatic Devices – Instrument Air | -10 | 7,500 |
Liquids Unloading – Controlled | 1 | 5,000 |
Centrifugal Compressors (Blowdown Valve) – LDAR | 2 | 12,500 |
Reciprocating Compressor Rod Packing – LDAR | 3 | 17,500 |
Pipeline Venting – Pump Down | 8 | 20,000 |
LDC Meters and Regulators – LDAR | 41 | 25,000 |
Note. Marginal abatement cost table for methane reductions by eight sources. Approximate values from ICF International for the Environmental Defense Fund (ICF International, 2015). EDF Terms of Use
Conclusion
In summary, the need for immediate reductions in CH4 emissions is imperative to limiting global warming and improvement of air quality (IEA, 2023). With the advancements in technology and satellite imaging, the ability to map global CH4 leakages has become more accessible. While there are some economic incentives to prevent methane leaks, there are not enough. Governments need to act now with a genuine effort to effect change. To have the possibility of global savings of roughly USD 470 billion if emissions are reduced (CACC & UNEP, 2021). As technology continues to advance, the use of satellites will be crucial in affecting the MAC and global CH4 emissions.
Media Attributions
Figure 1: “MethaneSAT’s algorithm and sensing technology can also detect the rate of methane emissions escaping from specific oil/gas sources” by MethaneSAT (n.d.), via Environmental Defense Fund, is used under the Environmental Defense Fund Terms of Use.
References
Ayasse, A. K., Dennison, P. E., Foote, M., Thorpe, A. K., Joshi, S., Green, R. O., Duren, R. M., Thompson, D. R., & Roberts, D. A. (2019). Methane mapping with future satellite imaging spectrometers. Remote Sensing, 11(24), Article 3054. https://doi.org/10.3390/rs11243054.
Black, R. (2014, September 9). Climate economics – costs and benefits. Energy & Climate Intelligence Unit. https://eciu.net/analysis/briefings/climate-impacts/climate-economics-costs-and-benefits.
Climate and Clean Air Coalition, & United Nations Environment Programme. (2021). Global methane assessment (summary for decision makers). https://www.ccacoalition.org/resources/global-methane-assessment-summary-decision-makers.
Cusworth, D. H., Jacob, D. J., Sheng, J.-X., Benmergui, J., Turner, A. J., Brandman, J., White, L., and Randles, C. A.: Detecting high-emitting methane sources in oil/gas fields using satellite observations, Atmos. Chem. Phys., 18, 16885–16896, https://doi.org/10.5194/acp-18-16885-2018, 2018
Edmond, C. (2023, February 2). Satellite tracking is helping scientists pinpoint the worst emissions offenders. World Economic Forum. https://www.weforum.org/agenda/2023/02/climate-emissions-satellite-tracking/.
Ehret, T., de Truchis, A., Mazzolini, M., Morel, J-M., d’Aspremont, A., Lauvaux, T., Duren, R., Cusworth, D., & Facciolo, G. (2022). Global tracking and quantification of oil and gas methane emissions from recurrent Sentinel-2 imagery. Environmental Science & Technology, 56(14), 9827–10546. https://doi.org/10.1021/acs.est.1c08575.
English, J. (2024, March 4). New satellite will track elusive methane pollution from oil and gas industry globally. CBC News. https://www.cbc.ca/news/climate/methanesat-launch-climate-1.7132770.
Hausman, C., & Raimi, D. (2019, February 4). Plugging the leaks: Why existing financial incentives aren’t enough to reduce methane. Kleinman Center for Energy Policy. https://kleinmanenergy.upenn.edu/research/publications/plugging-the-leaks-why-existing-financial-incentives-arent-enough-to-reduce-methane/
ICF International. (2015, October 5). Economic analysis of methane emission reduction opportunities in the Canadian oil and natural gas industries. Environmental Defense Fund. https://www.pembina.org/pub/economic-analysis-of-methane-emission-reduction-opportunities-canadian-oil-and-natural-gas.
International Energy Agency. (2023, February). Global methane tracker 2023. https://www.iea.org/reports/global-methane-tracker-2023.
Kayrros. (n.d.). Kayrros Methane Watch. Retrieved March 27, 2024, from https://methanewatch.kayrros.com/.
Nisbet, E. G., Fisher, R. E., Lowry, D., France, J. L., Allen, G., Bakkaloglu, S., Broderick, T. J., Cain, M., Coleman, M., Fernandez, J., Forster, G., Griffiths, P. T., Iverach, C. P., Kelly, B. F. J., Manning, M. R., Nisbet-Jones, P. B. R., Pyle, J. A., Townsend-Small, A., al-Shalaan, A., Warwick, N., & Zazzeri, G. (2020). Methane migration: Methods to reduce emissions, on the path to the Paris agreement. Reviews of Geophysics, 58(1), Article e2019RG000675. https://doi.org/10.1029/2019RG000675.
Olczak, M., Andris, P., & Christopher, J. (2020). Satellite and other aerial measurements: A step change in methane emission reduction? European University Institute. https://doi.org/10.2870/930570.
Pandey, S., Gautam, R., Houweling, S., Denier, H., Sadavarte, P., Borsdorff, T., Hasekamp, O., Landgraf, J., Tol, P., Van Kempen, T., Hoogeveen, R., Van Hees, R., Hamburg, S. P., Maasakkers, J. D., & Aben, I. (2019). Satellite observations reveal extreme methane leakage from a natural gas well blowout. Proceedings of the National Academy of Sciences, 116(52), 26376-26381. https://doi.org/10.1073/pnas.1908712116
Pison, I., Ringeval, B., Bousquet, P., Prigent, C., & Papa, F. (2013). Stable atmospheric methane in the 2000s: Key-role of emissions from natural wetlands. Atmospheric Chemistry and Physics, 13(23), 11609–11623. https://doi.org/10.5194/acp-13-11609-2013.
Saunois, M., Jackson, R. B., Bousquet, P., Poulter, B., & Canadell, J. G. (2016). The growing role of methane in anthropogenic climate change. Environmental Research Letters, 11(12), Article 120207. http://doi.org/10.1088/1748-9326/11/12/120207.