The Potential of Hydrogen Fuel Cell Vehicles
Zachary Johnson
Introduction
The era of hydrogen fuel cell vehicles (HFCV) is approaching soon as technological breakthroughs are occurring rapidly. Provided that HFCV produces zero emission, this short commentary aims to determine the emission and cost of producing hydrogen fuel cells (HFC). Additionally, due to hydrogen likely becoming a major fuel source, this commentary will address the costs and implications regarding the development of hydrogen fueling stations (HFS).
Hydrogen Fuel Cell Vehicles Production
Emissions
Currently, the supply chain of HFCs is driven by a variety of production methods. These methods include the use of fossil fuels such as natural gas reforming, coal gasification, the use of renewable sources of energy for electrolysis such as wind, solar, and hydroelectric, and nuclear reactors, including nuclear fission (Granovskii, 2006; Kothari et al., 2008). Globally, hydrogen (H2) production is dominated by using fossil fuel feedback systems (Kothari et al., 2008). The proportion of hydrogen produced directly using fossil fuels is 96%, while 4% is produced indirectly using electricity sourced from fossil fuels.
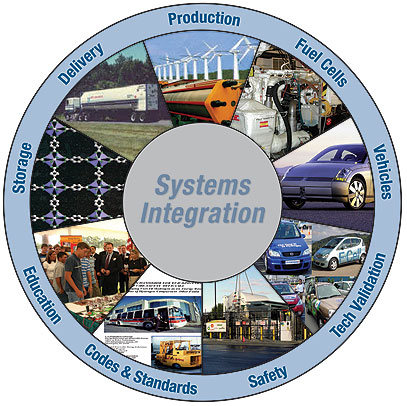
Studies have determined that hydrogen production through electrolysis using wind energy was a greater option for mitigating greenhouse gas emissions, while natural gas reforming showed greater pollution reduction (Granovskii, 2006). Moreover, producing H2 directly through fossil fuels produces between 7.33 and 29.33 kg of CO2 per kg of H2 when at 75% system efficiency. In contrast, H2 produced by electrolysis with the use of renewable energy sources had zero CO2 emissions (Kothari et al., 2008).
Moreover, if, on average, HFCV efficiency is 1 kg H2/100 km and the average person in Canada drives 20,000 km per year, then 200 kg of H2 is used per year, which equates to 1,466 to 5,866 kg of CO2 emitted H2 production using fossil fuels (Winkless, 2016).
For electric vehicles (EV), the average CO2 emitted from battery production is 100 kg equivalent per kilowatt hour (kWH) (Linder et al., 2023). The U.S. Department of Energy (2020) estimates that, when considering production and the lifetime of an EV, the annual CO2 emissions are equivalent to 1,237 kg per vehicle annually. When comparing internal combustion engines (ICE), the fuel economy for a gasoline engine is 8.9 L/100 km and for every litre of gasoline burned, 2.3 kg of CO2 is emitted. Lastly, in a year of driving 20,000 km, this is equivalent to 4,094 kg of CO2 annually per vehicle. A summary of the annual emissions is shown in Table 1.
Costs
The cost of producing H2 shows great variation, depending on the processing method. The cost for natural gas reforming (NGR) is 103.6 RS/kg, where RS is capital cost (USD 1 = 44 RS), which is equivalent to USD 2.4 / kg H2 (Kothari et al. 2008). Electrolysis varied depending on the energy source, and it was determined to be between 73.5 RS/kg (USD 1.67 / kg H2) and 854.3 RS/kg (USD 19.4/kgH2).
The annual cost of an HFCV would then be equal to CAD 480 per year to operate, assuming 200 kg of H2 is used per year and the method of production is NGR. In comparison, an EV uses approximately 15 kWH per 100 km, and a year’s worth of driving (20,000 km) is equal to 3,000 kWH. The cost of charging at Tesla stations is CAD 0.26 per kWH, and if 3,000 kWH are used annually, then it would cost $780 to operate (Energuide.be, n.d.). The average cost of regular gasoline is CAD 1.68/L, and in a year, an ICE would burn 1,780 L of gasoline, costing the operator CAD 2,990 annually. A summary of the annual operating cost of vehicles is displayed in Table 1.
Table 1: Comparing Annual Emissions & Costs Between Vehicle Types
Skip Table 1 | ||
Vehicle Type | Annual Emissions (kh CO2) | Annual Operator Cost (CAD) |
---|---|---|
Hydrogen Fuel Cell | 1,466 to 5,866 | 480 |
Electric | 1,237 | 780 |
Internal Combustion Engine | 4,094 | 2,990 |
Note. Annual emissions from the U.S. Department of Energy (2020). Annual operator costs from Kothari et al. (2008) and Energuide.be (n.d.).
Hydrogen Fuel Station Production
As of 2013, there were 224 HFS globally, with 43% in the Americas, 34% in Europe, and 23% in Asia (Alazemi and Andrews 2015). HFS is designed to either have H2 delivered to the station, produce H2 on-site, or even a combination of the two. HFS construction costs between USD 1.8 and 5.9 million in the U.S. (Richardson et al. 2015). Typically, the stations have fixed capacities of H2/day, such as 60 to 1,000 kg/day (Richardson et al., 2015; Aminudin et al., 2023). The average cost of an HFS is USD 2.4 million for 500 kg/day or USD 5.7 million for 1,000 kg/day; however, the cost will vary depending on the method of H2 production (Aminudin et al., 2023). HFS require expensive safety devices, which add to the cost, as hydrogen is very combustible, with a range of 7% to 45% in the air (Richardson et al., 2015). HFS’s high cost and lack of flexibility deter private investors, resulting in the financial burden belonging to governments.
Conclusion
In conclusion, as we move into the HFCV era, we can expect that HFC production and HFS will be extremely expensive. However, with enough traction and technological advancements, the costs may drop. It is recommended that HFCs be developed using electrolysis using hydrogen power as it’s the most cost-effective and environmentally efficient production method. Additionally, hydrogen fuel cell vehicles appear to be the best option compared to electric and ICE as they offer similar emissions to EVs and the lowest operating cost.
Lastly, due to the financial burden of developing HFSs falling on governments, it is recommended that they have incentives for the public to switch to HFCV. However, the implementation of a tax would not work as the tax rate would be extremely high to equal the marginal environmental damages (Sartzetakis & Tsagaris, 2005). Subsidization was also determined to be an insufficient option as the tax rate would be too low and not equal the marginal environmental damages. A possible incentive could be replacing old internal combustion technology with the new HFC technology. The government could subsidize the cost of switching technologies to account for vehicle depreciation. Lastly, we could convert gas stations into HFS to encourage the switch to HFCVs, although this was proven to have a low chance of success.
Media Attribution
Figure 1: “Hydrogen Program Systems Integration” by the Office of Energy Efficiency and Renewable Energy [an office of the U.S. Department of Energy] (n.d.), via Energy.gov, is in the public domain.
References
Alazemi, J., & Andrews, J. (2015). Automotive hydrogen fuelling stations: An international review. Renewable and Sustainable Energy Reviews, 48, 483–499. https://doi.org/10.1016/j.rser.2015.03.085.
Aminudin, M. A., Kamarudin, S. K., Lim, B. H., Majilan, E. H., Masdar, M. S., Shaari, N. (2023). An overview: Current progress on hydrogen fuel cell vehicles. International Journal of Hydrogen Energy, 48(11), 4371–4388. https://doi.org/10.1016/j.ijhydene.2022.10.156.
Energuide.be. (n.d.). How much power does an electric car use? Sibelga. Retrieved March 19, 2024, from https://www.energuide.be/en/questions-answers/how-much-power-does-an-electric-car-use/212/#:~:text=Most%20electric%20vehicles%20can%20cover,car%20reaches%2090%20%25%20and%20more.
Granovskii, M., Dincer, I., & Rosen, M. A. (2006). Environmental and economic aspects of hydrogen production and utilization in fuel cell vehicles. Journal of Power Sources, 157(1), 411–421. https://doi.org/10.1016/j.jpowsour.2005.07.044.
Kothari, R., Buddhi, D., & Sawhney, R. L. (2008). Comparison of environmental and economic aspects of various hydrogen production methods. Renewable and Sustainable Energy Reviews, 12(2), 553–563. https://doi.org/10.1016/j.rser.2006.07.012.
Linder, M., Nauclér, T., Nekovar, S., Pfeiffer, A., & Vekić, N. (2023, February 23). The race to decarbonize electric-vehicle batteries. McKinsey & Company. Retrieved March 18, 2024, from https://www.mckinsey.com/industries/automotive-and-assembly/our-insights/the-race-to-decarbonize-electric-vehicle-batteries.
Office of Energy Efficiency and Renewable Energy. (n.d.). Systems integration [Image]. U.S. Department of Energy. https://www.hydrogen.energy.gov/program-areas/systems-integration.
Richardson, I. A., Fisher, J. T., Frome, P. E., Smith, B. O., Guo, S., Chanda, S., McFeely, M. S., Miller, A. M., & Leachman, J. W. (2015). Low-cost, transportable hydrogen fueling station for early market adoption of fuel cell electric vehicles. International Journal of Hydrogen Energy, 40(25), 8122–8127. https://doi.org/10.1016/j.ijhydene.2015.04.066.
Sartzetakis, E. S., & Tsigaris, P. (2005). Environmental externalities in the presence of network effects: Adoption of low emission technologies in the automobile market. Journal of Regulatory Economics, 28, 309–326. https://doi.org/10.1007/s11149-005-3961-3.
U.S. Department of Energy. (2022). Alternative fuels data center: Emissions from electric vehicles. Retrieved from March 19, 2024, from https://afdc.energy.gov/vehicles/electric-emissions.
Winkless, L. (2016, June 1). Are hydrogen fuel cell cars becoming…normal? Forbes. Retrieved March 18, 2024, from https://www.forbes.com/sites/lauriewinkless/2016/06/01/are-hydrogen-fuel-cell-cars-becoming-normal/?sh=664b9f53683a.